P4 - Mechanical strategies to avoid interspecies competition in trypanosomes
Trypanosomes - omnipresent, scourge and biologcial model
African trypanosomes are probably one of the most extensively studied eukaryotic cells. For more than 120 years they have attracted scientists from very different disciplines. A plethora of trypanosome species parasitize basically all vertebrates, from fish to birds. Their fascinating biology has been detailed by many generations of evolution biologists, geneticists, cell and molecular biologists and, of course, parasitologists. Trypanosomes cause deadly diseases such as human sleeping sickness and nagana in cattle. Today, sleeping sickness is a prototypic neglected tropical disease of poverty, but the beginning of the last century has seen enormous outbreaks and trypanosomes were considered the scourge of Africa. In the past 50 years, Trypanosoma brucei has made a career as cell biological model. Many fundamental discoveries, such as GPI-anchoring of proteins or RNA-editing have been made in trypanosomes, probably because the parasites have evolved streamlined survival strategies using these processes.
Flagellum, flagellar pocket, and membrane flux
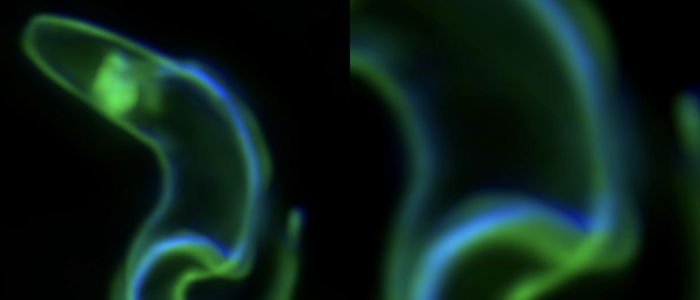
African trypanosomes have an elongated, spindle-shaped cell body to which a single flagellum is attached in a 180° turn. The more or less short free end of the flagellum, which protrudes beyond the cell body, shows a planar beat with a frequency of about 20 Hz. The flagellar wave propagates to the base of the flagellum, deforming the entire cell. Due to the highly asymmetric shape of the parasite, the planar beat translates into a corkscrew-like movement in the direction of the free end of the flagellum. The base of the flagellum is located within an enigmatic ‘extracellular’ organelle near the posterior pole of the cell, the flagellar pocket. On the one hand, the pocket is compartmentalised and closed to the outside, on the other hand, it is a place of tumultuous traffic. It is the only site for endocytosis and exocytosis, i.e. all membrane flux into and out of the cell takes place at this tiny site, which accounts for only 5% of the parasite surface. The membrane flux is not only localised but also surprisingly fast. We found that the entire cell surface is recycled once every 10 minutes, which means that about 15 μm2 of membrane must pass through the flagellar pocket per minute - in both directions.
Hydrodynamic sorting and antibody clearance
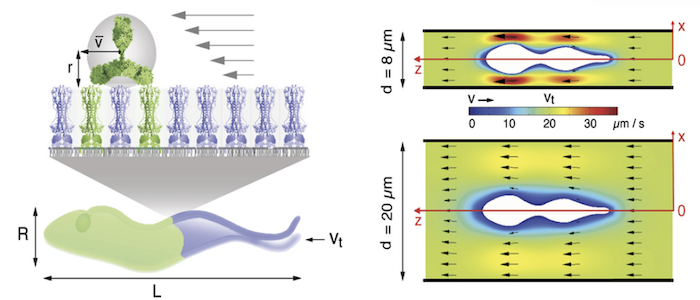
Why do the parasites invest so much in membrane recycling. The possible answer suggests a direct link between the cell design of the trypanosome (bauplan), the directional motility of the parasite and the rapid recycling of its cell surface: As obligate extracellular parasites, trypanosomes are under constant surveillance by the host immune system. The parasites are covered with a dense coat of variant surface glycoproteins (VSGs) that shields the plasma membrane and embedded invariant proteins from host antibodies. Trypanosomes have hundreds of VSG types, all of which have a similar structure but are antigenically very different. The parasites stochastically change the VSG type, i.e. they evade the immune response by changing their cell surface coat. This process of antigenic variation essentially continues until the host's defences break down, and death occurs after several weeks to months of infection. In an early stage of antibody response, the parasites use another strategy of immune evasion, namely antibody clearance. The incessant directional motility of the parasites creates a hydrodynamic flow on their cell surface. When antibodies bind to VSGs, the flow force drags the VSG-antibody complexes against the swimming direction towards the posterior pole of the cell. This is where the flagellar pocket is located and the rapid endocytosis rate ensures that the antibody-complexed VSGs are immediately endocytosed. While the antibody is destroyed in the lysosome of the pathogen, the VSG is recycled to the cell surface. Thus, in trypanosomes, motility and the resulting hydrodynamic drag force, are directly involved in the survival and persistence of the parasite. Thus, trypanosome antibody clearance can be considered a prime example of the importance of physics in parasitism.
Avoding Interspecies Competition by Mechanics
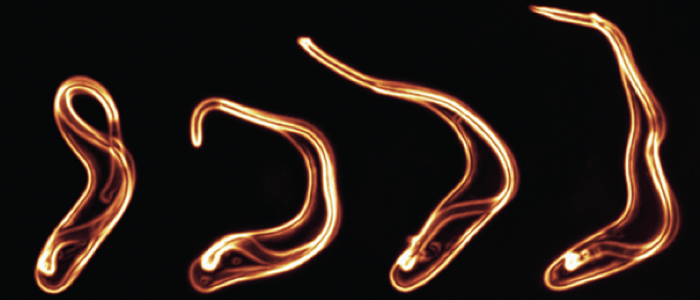
In recent years, we have detailed that not all trypanosome species have the same swimming behaviour and that their cell architecture also varies slightly. While Trypanosoma vivax is a very fast swimmer with a long and slender cell body, Trypanosoma congolense is a poor swimmer with a rather stumpy morphology. Trypanosoma brucei lies between these two trypanosome species. While T. vivax and T. brucei both respond to the presence of obstacles that mimic red blood cells with increased swimming speed and more persistent movements, this is not the case for T. congolense. We hypothesise that trypanosomes use their different mechanical properties and swimming abilities to occupy different infection niches in the host. All three species are transmitted from tsetse flies to potentially the same animal host. Physical adaptations might be involved in avoiding competition between phylogenetically related trypanosome species. Indeed, T. brucei is mainly found in tissue spaces, while T. vivax colonises the bloodstream and T. congolense the peripheral circulation of the host. Interestingly, T. congolense attaches to erythrocytes and blood vessel endothelia in a part of the circulation where the slowest blood flow prevails. The poor swimmer thus attaches to the walls of its niche, while the fast swimmers benefit from the presence of red blood cells and constricting geometries. PoP project 4 aims to test the hypothesis of "avoiding interspecies competition by mechancial adaptation".