About
Why studying the Physics of Parasitism?
Parasitism, the intimate antagonistic liaison between two species, has always fascinated scientists from a variety of disciplines. Traditionally, parasitology was concerned mainly with organismic studies, while today’s parasitology focuses on medically-relevant cellular and molecular mechanisms, at ever-increasing depth. The priority programme “Physics of Parasitism” defines a new frontier in this field, namely the physics of parasites interacting with their hosts. This interaction is controlled by the anatomy of the parasites (Bauplan), the physics of their locomotion, and the mechanics of their attachment to host structures. Parasitism has evolved many times and hence, there are numerous convergent solutions to the challenge of how to physically hijack a host. These long periods of co-evolution have equipped parasites with high degrees of optimality. Examples are parasitic tools such as suckers and shields, or refined locomotive devices that allow attachment and also navigation in various body fluids, in crowded and confined spaces, and in highly viscous environments - often at surprisingly high speeds. The priority programme studies the physics of parasitism using a selection of complementary and tractable parasites that colonise representative host niches. These include parasitic protists such as Plasmodium, Toxoplasma, and Trypanosoma, as well as metazoan parasites such as Schistosoma, Fasciola, and Echinococcus. We ultimately aim to elaborate a comparative and quantitative framework of the physical constraints and mechanical forces acting at the dynamic parasite-host interfaces. We will measure the material properties and mechanics of parasites in their niches, uncover the physical basis of their locomotion, and determine the mechanical and physical basis for their attachment. To reach these goals, the priority programme uniquely combines expertise from parasitology, molecular cell biology, experimental and theoretical physics, mathematics and simulation science. In this way, “Physics of Parasitism” opens new chapters in both parasitology and the physics of life. We envisage that the results obtained during our interdisciplinary endeavour might expose novel ways of combating parasitic diseases based on mechanobiology, against which resistances are unlikely to evolve.
The Bauplan of parasites often represents extreme solutions for tuning cell or body elasticity and motion. Some parasites construct exceptionally elastic cytoskeletal corsets that combine an enormous tensile flexibility with high mechanical stability. Other parasites have evolved suckers and many additional tools with which to attach to their hosts. Many parasites undergo complex life cycles, in which they sequentially develop adapted variations of a basic Bauplan. Some exhibit various stages that look similar at first sight, but which have very different mechanical properties and motion behaviours, all based on restructuring of cytoskeletal and membrane structures. In addition, the life cycle of some parasites involves the formation of extremely tough cysts, similar to bacterial spores, although some parasites can be highly proliferative within cysts (e.g. Plasmodium oocysts). Very little is known about the material properties of such stages.
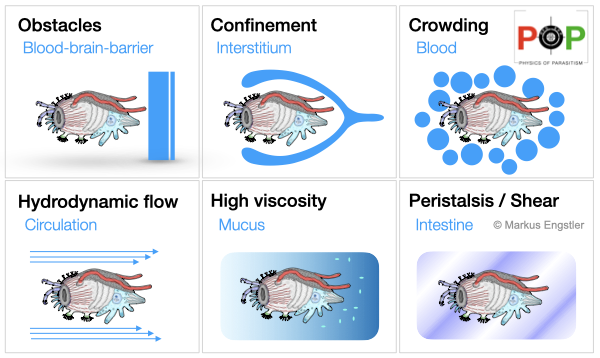
In contrast to free-living organisms, which are exposed to dynamic and unpredictable environments, parasites tend to occupy extremely specific niches with clearly defined properties, but which are nonetheless hostile to their presence and which they often can use only for a limited amount of time. Parasites are always under attack from their hosts, and have to operate in a constant defence mode. They need to cope with all levels of the immune response and have to withstand multiple mechanical challenges, fluid flow, peristalsis, barriers, crowding and confinement.
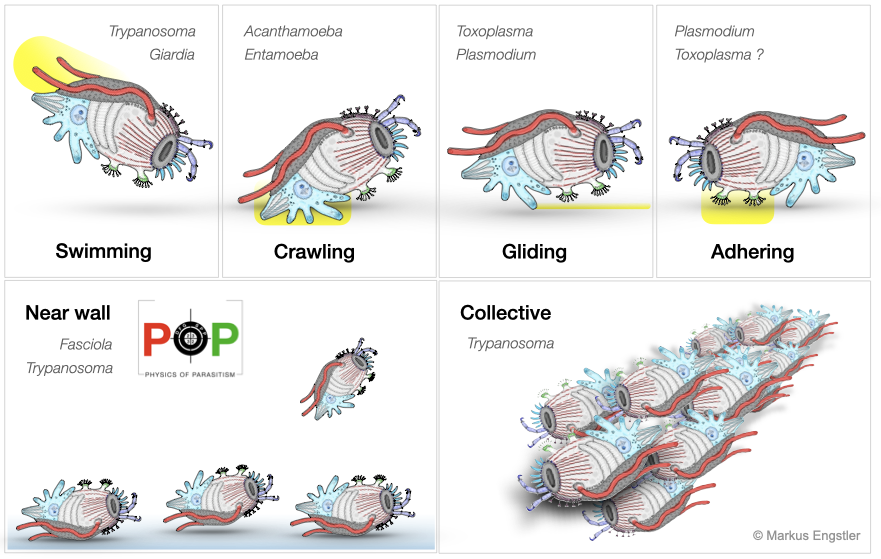
The journey towards their consecutive host niches requires refined locomotion strategies. Parasites can migrate passively (e.g. with the blood flow), or actively, by swimming, crawling or gliding - which is especially important when barriers have to be crossed, flow is encountered, or the elastic properties of the environment change. While undergoing free locomotion, many parasites have to cope with viscoelastic environments such as the intestinal mucus. In all cases, the physical conditions of the environment define strong restrictions for how propulsive forces have to be generated by the molecular machines of parasites, especially in the face of external and internal friction and noise. Although parasite motility is essential for survival, the actual modes of parasite locomotion have long been neglected, and only recently has the impact of changing host micro-environments on parasite motility been elucidated, especially by members of the PoP consortium .
Microscopic parasites experience motion in the world of low Reynolds numbers. Viscosity dominates, and inertia plays hardly any role, meaning that hydrodynamics is dominated by laminar and creeping flows. With every change of shape, the parasite transmits forces to the surrounding liquid and fluid flows, according to the Stokes equation. The flows in turn set the parasites in motion by exerting reaction forces and torques. Following Purcell's scallop theorem, parasites and similarly-sized objects can only move if this shape change is non-reciprocal. Swimming and steering of flagellated cells have been experimentally studied mainly with bacteria, sperm, and algae. Predictions include surface attraction due to hydrodynamic and steric interactions, as well as steering and navigation mechanisms due to variation of the flagellar shape and dynamics. For most microbes, these non-reciprocal shape changes are either achieved by employing rotating flagella (many bacteria), or flexible cilia with shapes that vary during the beating cycle (many algae). Parasites have evolved more complex shape changes that are difficult but rewarding to analyse in terms of hydrodynamics.
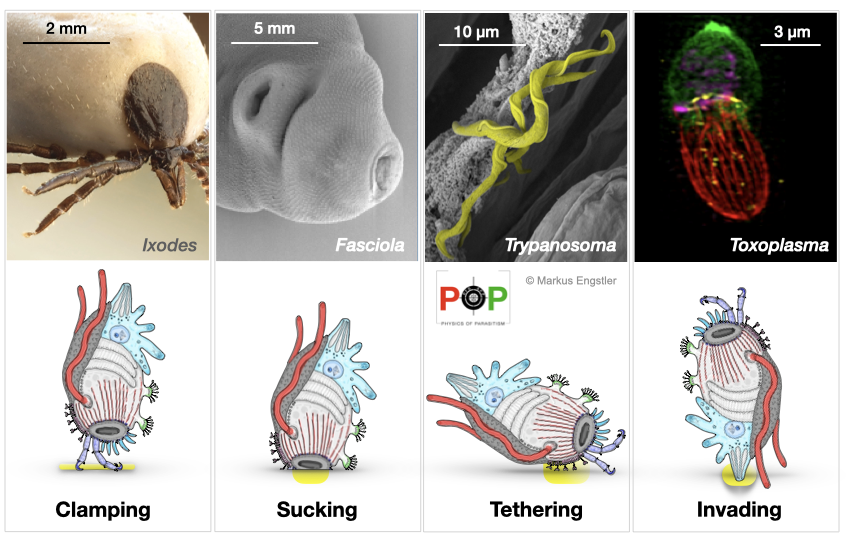
Attachment to solid substrates is a key requirement for nearly all parasitic organisms. The actual modes of attachment that parasites depend on are highly diverse ranging from proteinaceous ligand-receptor interactions that appear to generate forces in the range of tens of pN to mechanical systems such as hooks, locks, clamps and spacers that can generate mN. Thus, forces can apparently span 6 orders of magnitude, and it is unclear exactly which physical theory applies to each scale range. The nature of the prevailing force (e.g. mechanical, electrostatic, chemical, van der Waals, capillary or viscous) also highly depends on the physical conditions during attachment, e.g., whether it involves dry matter (e.g. at the skin) or happens in the wet or even under immersed conditions (e.g. in host tissue). Through judicious selection of parasites, PoP members aim to analyse attachment mechanisms at highly different force scales and will develop the respective theoretical framework. By comparing attachment modules of parasites from different branches of the tree of life, PoP members will identify physical homologues of ‘micromachines’ that, possibly, evolved along very different molecular paths.